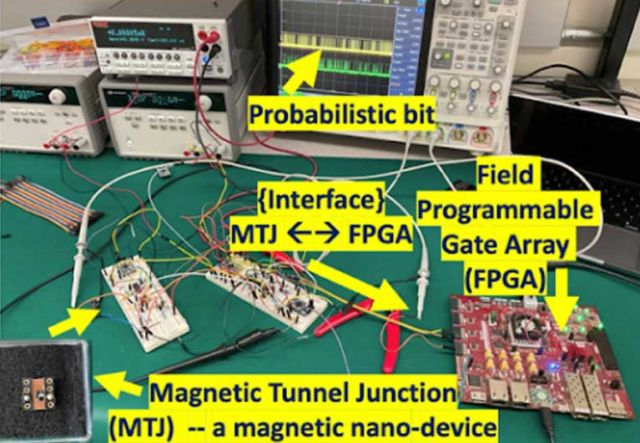
ECE Research Initiative
Kerem Çamsarı, Orchestrating Physics for Unconventional Systems (OPUS) Lab | "Delivering Hardware Advances for the Beyond-Moore Era of Electronics"
From The ECE Current Newsletter (Fall 2022)
Çamsari believes the beyond-Moore era of electronics will be defined by a creative combination of heterogeneous computing systems, blurring traditionally separate layers of abstraction from Physics to Systems
The field of electronics has been driven by Moore’s Law for at least six decades. In the last decade or so, transistor and its modern variants have reached atomic dimensions and it is very difficult to continue scaling them. Meanwhile, enormous algorithmic advances in the field of Machine Learning and Artificial Intelligence (AI) have recently taken over the world. The success of "deep learning" led to larger and larger neural networks achieving impressive feats. We have now chatbots that can write newspaper columns or digital AI artists that can design magazine covers from simple English prompts. While these are incredibly impressive, there is an enormous cost behind these AI models in the energy, resources, and time they use.
Given these insatiable computational demands in AI, a new thread of research with a steady following has emerged. The basic idea is to augment traditional computing hardware with new and emerging materials and phenomena by connecting new physics to the needs of algorithms and applications. This approach is sometimes summarized by the credo: "Let physics do the computing." In fact, this is a good description of the related field of quantum computing (QC): "QC is all about building computers out of quantum building blocks to solve "naturally quantum" problems, for example, quantum chemistry and solving the quantum many-body problem. In a similar vein, my lab is leading the development of probabilistic computers with p-bits, naturally suited to implement probabilistic algorithms and functionality. Probabilistic thinking is very common in modern AI and we believe p-bits may play an important role in energy-efficient, next-generation hardware for AI and computing.
As an example of this Physics-to-Systems approach we follow in our lab, consider Figure 1. Here we make use of the natural noise encountered in magnetic nanodevices to build energy-efficient probabilistic bits to use them in conjunction with existing technology. Such ideas often require a deep understanding of the underlying algorithms to adjust them in hardware-friendly designs, which is the co-design aspect mentioned earlier. Another example of this thinking is our algorithm-architecture co-design result that recently appeared in Nature Electronics (Aadit et al., 2022). In that paper, we showed how modifying well-known algorithms to make them "sparse" and hardware-friendly could lead to massive parallelism, with substantial improvements in time and energy to solution for hard computational problems.
Due to the interdisciplinary nature of this field, we are interested in various layers of abstraction in computing, from physics and devices all the way up to architectures and algorithms. We often employ what is known as co-design techniques where requirements in one layer of abstraction (e.g., devices) dictate how another layer (e.g., algorithms) should be modified. We spend a lot of time thinking how to combine different domains smoothly to one another. This makes our research highly interdisciplinary and students enjoy the all-around understanding they have to develop to be creative in this field.
Going further, we believe scaling up novel CMOS + X style hardware where traditional CMOS hardware is enhanced with new and emerging technologies (X= magnetism, ferroelectricity, etc.), through heterogeneous integration in monolithic architectures and other methods, will eventually lead to success stories in the beyond-Moore era of electronics. At least this is our hope and vision for a more sustainable and equitable future in computing.
Çamsarı's Orchestrating Physics for Unconventional Systems (OPUS) Lab